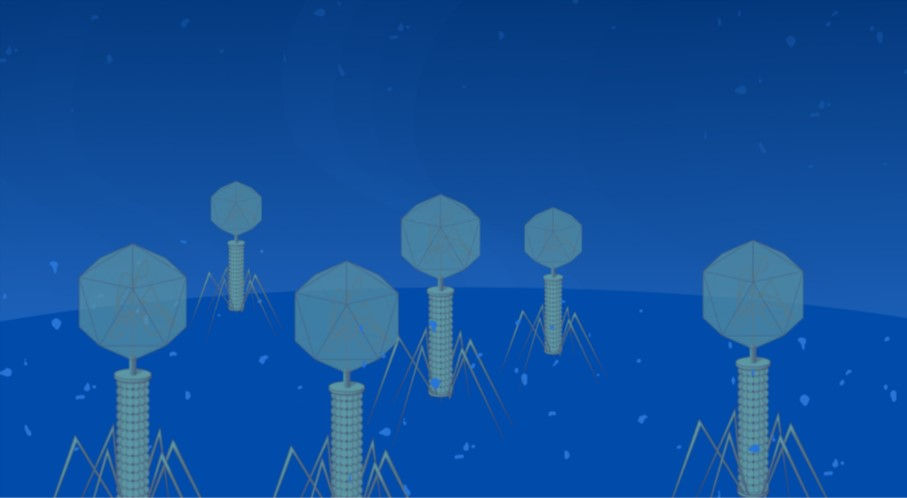
Togo picture gallery maintained by Database Center for Life Science (DBCLS) (https://creativecommons.org/licenses/by/3.0/deed.en)
Ever since the discovery of antibiotics in the early 20th century, iconic drugs such as Penicillin have served at humanity’s side as steady guardians against bacterial infections. They have saved countless lives and played a great role in increasing human life expectancy by decades (Adedeji, 2016). However, the treasure of antibiotics may be set to tarnish in the near future due to one phenomena: antibiotic-resistant bacteria.
Bacteria exhibit high rates of mutation compared to other organisms. Most of these mutations are not significant, but in extremely rare instances, they can confer antibiotic resistance onto the bacteria. For example, a lucky mutation could allow the microbes to produce enzymes that inhibit the action of the drugs, or break them down entirely (CDC, 2020).
Unfortunately, the use of antibiotics inadvertently leads to resistance: because antibiotics are so effective against normal bacteria, the only germs that aren’t wiped out by the drugs are those which have mutated and become antibiotic-resistant. This new bacterial strain can then reproduce rapidly, dividing and spreading to other hosts. With enough time and random chance, superbugs with resistance to almost all antibiotics can emerge. Unsafe medical practices such as overprescribing antibiotics have accelerated the advent of these multi-drug resistant bacteria superbugs, leading to hundreds of thousands of deaths (Munita & Arias, 2016).
As a solution to this crisis, scientists are constantly searching for new antibiotics or similar medicines that bacteria aren’t yet resistant to. One such medicine involves fighting fire with fire: using pathogens to kill other pathogens. This medicine, which could be humanity’s saving grace in the face of genetically impervious mega-germs, is known as phage therapy. Discovered more than 100 years ago, phage therapy involves using a special type of virus called bacteriophages (phage being Latin for “eater”) to eliminate infections.
Until recently, bacteriophage research has been put on the back burner of Western studies, mostly because there was no need for them; with antibiotics filling the same niche as phages, no company wished to spend the resources on pioneering the initial research or regulations. In the past decade, however, due to antibiotic resistance, phages have been thrust into the limelight of the medical world. In 2009, United Kingdom researchers administered serums containing 12 types of bacteriophages to 24 patients with chronic, antibiotic-resistant ear infections. The patients’ inflammation levels, self-reported pain, and quantity of bacteria in the infection all improved over the course of 42 days, with no negative side effects (Wright et al, 2009). This was the first controlled clinical trial of the 21st century that suggested phages are effective against superbugs. More recent studies have been performed in regards to burn infections and gastrointestinal diseases with similar results: phage therapy improved the conditions of all patients (Jault et al., 2019; Gindin et al., 2020). In one particularly encouraging study, researchers used experimental bacteriophages to treat a diabetic patient with a critical Acinetobacter baumannii pancreatic infection. A. baumannii is one of the most resistant strains of bacteria, and its victim lay dying at the beginning of the treatment, despite having received four-month-long antibiotics courses (Schooley et al., 2017). In a last-resort endeavor to save the man, doctors injected bacteriophage viruses directly into his pancreas. Within a few weeks, the patient made a miraculous recovery. Where antibiotics failed, phage therapy triumphed.
If phages have been proven fruitful in clinical settings, how exactly do they work? After bacteriophages find their way towards a bacterium by chance, they bind to specific receptors on the bacterium’s surface in a process called adsorption. Different bacteriophages are capable of binding to different receptors, and individual species have evolved to be specific to the receptors located on only a few closely related bacterial strains (Gordillo & Barr, 2019). After adsorption, the virus injects its DNA through the bacterium’s outer membrane. This DNA is immediately transcribed and translated by the cell’s ribosomes, creating a set of proteins dubbed the “early proteins.” These proteins’ purpose is to prepare for replicating the entire viral genome, and includes enzymes that repair the hole in the bacterial membrane; enzymes that degrade the host’s DNA for resources; and a DNA polymerase protein that creates DNA. The entire set of phage DNA is then replicated many times by the corresponding early proteins, leaving bunches of viral genome floating in the cytoplasm. In the next step, the “late proteins” are created in the same way as the early proteins. Among these are structural proteins that make up the tails and capsids (heads) of the viruses. After all of these parts are constructed, they are assembled into hundreds of fully functioning phages. The newly created viruses then secrete enzymes that lyse, or rupture, the cell from the inside. This releases the viral parasites to infect more bacteria, completing the cycle. (Todar, 2020)
A possible concern with phage therapy is that bacteria will simply continue to evolve resistance to phages, just as they do today with antibiotics. While phage therapy might still be useful, this would just push back the problem of resistance and force scientists to continuously look for new phage strains. However, phages are more effective than antibiotics in the long term because the viruses can continually adapt and evolve in response to the bacteria’s own developing resistance. This “arms race” between phages and bacteria has been ongoing for eons, and it has been proven that phages can mutate at a rapid enough rate to keep up with the bacteria (Gordillo & Barr, 2019). This is in contrast to antibiotics, which are typically derived from slowly-evolving fungi. Furthermore, even if bacterial strains become resistant to phages through widespread use of phage therapy, their antibiotic resistance would greatly decrease as a result (Oechslin, 2018). Thus, the use of both antibiotic and phage treatments could trap bacteria in a catch-22 situation.
Rapid mutation marks phage therapy as a great possible antibacterial medicine. Besides this characteristic, phage therapy is favorable over other treatments for the following reasons:
Antibiotics are comparable to carpet bombs, destroying all bacteria they come into contact with. On the other hand, phages are precise, targeted missiles: each species is able to bind to receptors on only a few bacterial strains. Thus, they do not disrupt the “good” bacteria in our bodies, such as the gut microbiome (Lin et al., 2017).
Phage therapy is self-limiting (Gordillo & Barr, 2019). Viruses require living hosts to reproduce: thus, after the bacterial infection has been quelled, the victorious bacteriophages will naturally die and cease to exist within the body.
Bacteriophages are extremely versatile. Scientists estimate that there are about 1031 phages in the world, more than every other organism combined (LaFee & Buschman, 2017). Doctors can exploit the extraordinary genetic diversity and abundance of phages to produce a wide variety of drugs (Gordillo & Barr, 2019).
Many bacteria are able to produce biofilms, densely packed groups of microbes that can cooperate in complex ways. While antibiotics have difficulty penetrating biofilms, phages are much more effective at eliminating these colonies (Srisuknimit, 2018).
However, every treatment has drawbacks, and phage therapy is no exception.
Firstly, bacteriophages’ specificity is a great weakness as well as a strength. Because the viruses infect only a few species of bacteria each, doctors must know the exact strain of bacteria attacking a patient before administering phage therapy (Lin et al., 2017). This requires valuable resources and delays the patients’ treatment, which could be deadly.
Phages are tricky to produce. The viruses are “farmed” by infecting large quantities of bacteria with a few phages and allowing them to burn through the colony and reproduce. If the isolation of these viruses from the bacterial corpses isn’t perfect, the immune system could react against the dead cells, triggering sepsis (Srisuknimit, 2018).
As foreign viruses, bacteriophages elicit immune responses such as inflammation, although to a much lesser extent than antibiotics. Due to lack of research, the exact interactions of the body’s immune system and bacteriophages remain unclear (Cisek et al., 2017).
Interestingly, some scientists have proposed techniques for using bacteriophages as a treatment for COVID-19. For one, phages can treat the secondary bacterial infections, such as pneumonia, that arise from the coronavirus (Omolo et al., 2020). Furthermore, doctors can use bacteriophages to create artificial antibodies against SARS-CoV-2 through a technique called phage display. Genes encoding antibodies that fight against the coronavirus are inserted into phages’ DNA, causing them to produce the antibodies and “display” them on their outside surfaces (Somasundaram et al., 2020). These antibodies can then be isolated and given to victims of the disease. However, this technique is still very experimental and imperfected (Wojewodzic, 2020). Lastly, phage capsids can be specially engineered to target and adhere to the binding sites of other viruses. With these binding sites blocked, the viruses are unable to attach to cells, preventing infection (Omolo et al., 2020). While this treatment has proven efficacious against influenza viruses, it still needs more extensive testing before being applied to the COVID-19 pandemic.
Overall, phage research and development has picked up speed in the last few years. Organizations such as Phages for Human Applications Group Europe (PHAGE) and Phages for Global Health have recently been founded to advance research into phage therapy and bring new phage treatments into the developing world (Moelling et al., 2018). As a first step towards concrete regulations, the FDA and European Commission have both funded continuing projects to assess the general safety of bacteriophages (Górski et al., 2018). Many experimental treatments and research studies by private companies are currently underway to develop phage therapy and create new medicines (Srisuknimit, 2018). However, antibiotic resistance will only continue to grow and accelerate with time. In the coming years and decades, we need to be ready for our first lines of defense against bacteria to fragment. Phage therapy is one of the most promising ways humanity can retain its dominance over disease, and prevent a collapse back to a time when death could follow from just a dirty cut or scrape. Thus, it is essential to put more of our resources into understanding bacteriophages and unlocking their full medical potential.
References
Adedeji W. A. (2016). THE TREASURE CALLED ANTIBIOTICS. Annals of Ibadan postgraduate medicine, 14(2), 56–57.
Center for Disease Control (2020). About Antibiotic Resistance. CDC, https://www.cdc.gov/drugresistance/about.html
Cisek, A. A., Dąbrowska, I., Gregorczyk, K. P., & Wyżewski, Z. (2017). Phage Therapy in Bacterial Infections Treatment: One Hundred Years After the Discovery of Bacteriophages. Current microbiology, 74(2), 277–283. https://doi.org/10.1007/s00284-016-1166-x
Gindin, M., Febvre, H. P., Rao, S., Wallace, T. C., & Weir, T. L. (2019). Bacteriophage for Gastrointestinal Health (PHAGE) Study: Evaluating the Safety and Tolerability of Supplemental Bacteriophage Consumption. Journal of the American College of Nutrition, 38(1), 68–75. https://doi.org/10.1080/07315724.2018.1483783
Gordillo Altamirano, F. L., & Barr, J. J. (2019). Phage Therapy in the Postantibiotic Era. Clinical microbiology reviews, 32(2), e00066-18. https://doi.org/10.1128/CMR.00066-18
Górski, A., Międzybrodzki, R., Łobocka, M., Głowacka-Rutkowska, A., Bednarek, A., Borysowski, J., Jończyk-Matysiak, E., Łusiak-Szelachowska, M., Weber-Dąbrowska, B., Bagińska, N., Letkiewicz, S., Dąbrowska, K., & Scheres, J. (2018). Phage Therapy: What Have We Learned?. Viruses, 10(6), 288. https://doi.org/10.3390/v10060288
Jault, P., Leclerc, T., Jennes, S., Pirnay, J. P., Que, Y. A., Resch, G., Rousseau, A. F., Ravat, F., Carsin, H., Le Floch, R., Schaal, J. V., Soler, C., Fevre, C., Arnaud, I., Bretaudeau, L., & Gabard, J. (2019). Efficacy and tolerability of a cocktail of bacteriophages to treat burn wounds infected by Pseudomonas aeruginosa (PhagoBurn): a randomised, controlled, double-blind phase 1/2 trial. The Lancet. Infectious diseases, 19(1), 35–45. https://doi.org/10.1016/S1473-3099(18)30482-1
Lafee, S., & Buschman, H. (2017). Novel Phage Therapy Saves Patient with Multidrug-Resistant Bacterial infection. UC Health—UC San Diego, https://health.ucsd.edu/news/releases/Pages/2017-04-25-novel-phage-therapy-saves-patient-with-multidrug-resistant-bacterial-infection.aspx
Lin, D. M., Koskella, B., & Lin, H. C. (2017). Phage therapy: An alternative to antibiotics in the age of multi-drug resistance. World journal of gastrointestinal pharmacology and therapeutics, 8(3), 162–173. https://doi.org/10.4292/wjgpt.v8.i3.162
Moelling, K., Broecker, F., & Willy, C. (2018). A Wake-Up Call: We Need Phage Therapy Now. Viruses, 10(12), 688. https://doi.org/10.3390/v10120688
Munita, J. M., & Arias, C. A. (2016). Mechanisms of Antibiotic Resistance. Microbiology spectrum, 4(2), 10.1128/microbiolspec.VMBF-0016-2015. https://doi.org/10.1128/microbiolspec.VMBF-0016-2015
Oechslin F. (2018). Resistance Development to Bacteriophages Occurring during Bacteriophage Therapy. Viruses, 10(7), 351. https://doi.org/10.3390/v10070351
Omolo, C. A., Soni, N., Fasiku, V. O., Mackraj, I., & Govender, T. (2020). Update on therapeutic approaches and emerging therapies for SARS-CoV-2 virus. European journal of pharmacology, 173348. Advance online publication. https://doi.org/10.1016/j.ejphar.2020.173348
Schooley, R. T., Biswas, B., Gill, J. J., Hernandez-Morales, A., Lancaster, J., Lessor, L., Barr, J. J., Reed, S. L., Rohwer, F., Benler, S., Segall, A. M., Taplitz, R., Smith, D. M., Kerr, K., Kumaraswamy, M., Nizet, V., Lin, L., McCauley, M. D., Strathdee, S. A., Benson, C. A., … Hamilton, T. (2017). Development and Use of Personalized Bacteriophage-Based Therapeutic Cocktails To Treat a Patient with a Disseminated Resistant Acinetobacter baumannii Infection. Antimicrobial agents and chemotherapy, 61(10), e00954-17. https://doi.org/10.1128/AAC.00954-17
Somasundaram, R., Choraria, A., & Antonysamy, M. (2020). An approach towards development of monoclonal IgY antibodies against SARS CoV-2 spike protein (S) using phage display method: A review. International immunopharmacology, 85, 106654. https://doi.org/10.1016/j.intimp.2020.106654
Srisuknimit, V., (2018). Fighting Fire with Fire: Killing bacteria with virus. Science in the News: Harvard University, http://sitn.hms.harvard.edu/flash/2018/bacteriophage-solution-antibiotics-problem/
Todar, K. (2020). Bacteriophage. Todar’s Online Textbook of Bacteriology, http://textbookofbacteriology.net/phage.html
Wright, A., Hawkins, C. H., Anggård, E. E., & Harper, D. R. (2009). A controlled clinical trial of a therapeutic bacteriophage preparation in chronic otitis due to antibiotic-resistant Pseudomonas aeruginosa; a preliminary report of efficacy. Clinical otolaryngology : official journal of ENT-UK ; official journal of Netherlands Society for Oto-Rhino-Laryngology & Cervico-Facial Surgery, 34(4), 349–357. https://doi.org/10.1111/j.1749-4486.2009.01973.x
Wojewodzic, M. W. (2020). Bacteriophages Could Be a Potential Game Changer in the Trajectory of Coronavirus Disease (COVID-19). PHAGE, http://doi.org/10.1089/phage.2020.0014
Written by Alex Borengasser
Edited by Devanandh Murugesan
Graphics by Tiya Shah
Group advised by Lakshmi Sriram
Comments